Single-Cell Analysis: An Overview
- 17th September 2021
- Posted by: Breige McBride
- Categories: Metabolomics, Proteomics, Single Cell Analysis
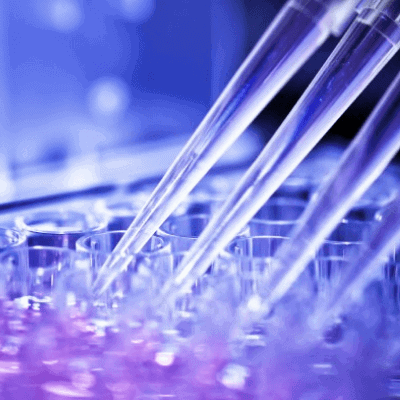
What is Single-Cell Analysis?
Single-cell analysis studies different aspects of the cell at an individual cell level. During single-cell analysis we can analyse ‘omics data (from areas such as the genome, proteome, transcriptome and metabolome). Single cells allow us to see mechanisms that we would lose when studying a large population of cells.
Multicellular organisms, by their nature, have cell-to-cell variation. From muscle cells to blood cells, and skin cells to cone cells in the eye, the human body has many diverse cell types with different functions and gene expression profiles. There are even heterogeneous cells within tissues that have subtle differences compared to others.
Often when researchers base gene expression measurements on a homogenised cell population from a tissue, they find misleading averages. The information from the entire cell population may not account for small changes in individual cells, which could be critical. Cells can differ in expressed protein levels and RNA transcripts, which may hold the keys to unanswered questions in many fields.
Using single-cell approaches allows for understanding in how cell networks work to coordinate responses on cell-population side levels.
Collecting Single Cells For Analysis
There are several ways that we can isolate a single cell from a population, such as via serial dilution, laser capture microdissection, and manual picking.
‘Hydrodynamic-based microfluidic biochips’ are another method to isolate single cells. Hydrodynamics uses mechanical barriers to separate the target cell from the main sample. After separation, researchers keep the target cells in a trapping site for analysis and investigation. The biochips use micro-level dimensions of flow channels in order to separate the cells.
Why Is Single-Cell Analysis Important?
Since single-cell analysis enables the study of cell-to-cell variation, it has many applications. Research into cancer, drug resistance, and ageing can all benefit from single-cell analysis. Microbiologists, immunologists, and neurologists also use single-cell analysis and it is popular in the fields of prenatal diagnosis and plant biology as well. Data from single-cell RNA Sequencing, better known as scRNA-seq, is able to tell us:
- Which cell types or states exist in a sample
- Which gene signatures and biomarkers distinguish the cell population
- What the differences are in cell-type composition between different individuals or conditions
- What the differences are in cell states between conditions
- How cells transition between states during development
- How cell types interact
Genomics – Single-Cell Benefits
The genome is the genetic material of an organism – DNA in humans. More than just genes (the coding regions of DNA), it also includes non-coding DNA and the genetic material found in mitochondria (in animals) and chloroplasts (in plants).
There are two main applications to studying the genomics of single-cell
- Tracking changes that occur in a bacterial population
- Studying the genetic evolution of cancer
In bacterial populations, we see phenotypic differences, and researchers often overlook the differences between bacteria cells by using bulk sequencing techniques. When studying the genetic evolution of cancer, which is constantly mutating, researchers observe alterations using the single-cell sequencing method.
How Does Single-Cell Genomics Work?
To study the genome on a single-cell level, we need to employ whole genome amplification strategies. We can use a number of these, such as
- Degenerate oligonucleotide-primed polymerase chain reaction (DOP-PCR)
Using the PCR method, DOP-PCR amplifies the entire genome of the cell using a large set of various primers. While simple to use, there is a lack of coverage of the whole genome.
- Multiple displacement amplification (MDA)
MDA uses random primers with a high-fidelity enzyme to amplify larger fragments of the genome and give greater coverage. High-fidelity enzymes allow for accurate replication of the genetic template, meaning that there are fewer errors and a true replication of the target code. MDA does still have sequence dependant bias, where certain sections of the genome are amplified at a greater level than others, purely due to their sequence.
- Multiple annealing and looping-based amplification cycles (MALBAC)
MALBAC are a method that aims to avoid the bias shown by DOP-PCR and MDA. MALBAC only copy genomic information of the original strand of DNA, instead of copying DNA copies. The downside to MALBAC is that it is less accurate than the two previous techniques, due to the enzyme used to copy the DNA strand.
Transcriptomics – Single-Cell Benefits
The transcriptome contains all RNA (including messenger RNA) molecules in the sample of cells. RNA molecules are expressed through transcription from the genes of an organism.
How Does Single-Cell Transcriptomics Work?
Transcriptomics on a single-cell level determines which genes are being expressed in the cell. Researchers will often use the transcriptome instead of the proteome for quantifying gene expression as there can be difficulties in amplifying cell protein levels. Transcriptomics does not take into account any post-transcriptional regulation, which means it is less accurate at determining actual gene expression when compared with proteomics.
Gene expression is studied via transcriptomics for three reasons:
- Gene dynamics
- RNA splicing
- Cell typing
Gene dynamics studies determine what changes in gene expression cause different cell characteristics e.g. during the development of an embryo. RNA splicing studies aim to understand how different transcript isoforms are regulated.
Cell typing studies identify different cells by the genes that they are expressing. Therefore, we can identify cells even when other known genetic markers are not present.
Techniques for studying single-cell transcriptomics are similar to those used for single-cell genomics. The transcriptome first needs to be quantified; this happens by converting RNA to complementary DNA (cDNA). This conversion is done using reverse transcriptase, which allows for the contents of a cell to be sequenced using next-generation sequencing methods (as used for single-cell genomics analyses). There is not enough cDNA present in a single cell for sequencing, so DNA amplification takes place to ensure that there is more cDNA and enables sequencing to occur.
Alternatively, researchers can attach fluorescent compounds to RNA hybridisation probes. They can then use these compounds to identify specific sequences. The sequential application of different RNA hybridisation probes allows for a full view of the single-cell transcriptome to be created.
Proteomics – Single-Cell Benefits
The proteome is the complete set of proteins that can be expressed by a cell or population of cells at a given time. Proteins can control how a cell acts and how gene expression can change through different stimuli. Proteomics is the study of proteins in how a cell operates; while similar to transcriptomics, proteomics takes into account post-transcriptional regulation and so is more accurate at determining gene expression.
How Does Single-Cell Proteomics Work?
There are two main approaches when studying proteomics at the single-cell level: antibody-based and mass spectroscopy-based methods.
Antibody-Based Methods
The main antibody-based methods use antibodies to bind to the relevant proteins. The antibodies used are also bound to fluorescent molecules or isotopes. Fluorescent molecules can be detected by the light they emit, while the isotopes can be shown through mass spectrometry. Different isotopes can be bound to different antibodies, which allows for multiple proteins in a single cell to be identified at once.
A secondary antibody-based method converts protein levels into DNA levels. Protein to DNA conversion allows for amplification of protein levels and next-generation sequencing to quantify these proteins. For conversion to occur, two antibodies are designed for each protein to be quantified. Each of the antibodies then has complementary single-stranded DNA attached; when the two antibodies bind to a protein, the complementary strands recombine and produce a piece of double-stranded DNA that is able to be amplified through PCR. The antibodies designed for each protein are individual and tagged with different sequences of DNA. Once amplified through PCR, the DNA can be sequenced which allows for the protein levels to be quantified.
Mass Spectroscopy-Based Methods
Mass spectroscopy-based methods to study a single-cell proteome have three key steps for peptide identification:
- Sample preparation
- Separation of peptides
- Identification of peptides
Sample preparation usually revolves around the specific cells chosen to study the proteome. Oocytes (immature egg cells/ova) and early cleavage-stage cells are large and provide enough material for researchers to use for analysis.
We can separate and isolate peptides in a number of ways. We can use reagents and centrifuging steps, magnetic beads, or filters to separate peptides out based on their characteristics. To separate proteins of different sizes, capillary electrophoresis or liquid chromatography are two methods that can be used. These allow the peptides to be ordered before quantification.
Peptide identification on a single-cell level uses similar methods to the identification of a larger level, with modifications needed purely for the small sample volumes. Researchers analyse mass spectroscopy data and compare it with information about peptides identified to protein quantification levels.
Metabolomics – Single-Cell Benefits
The metabolome is the total number of metabolites present in a cell or population of cells. Metabolites are the small molecules that are necessary for cellular metabolism and are usually intermediary instead of final products. Metabolomics, therefore, is the study of the chemical processes which include those metabolites.
How Does Single-Cell Metabolomics Work?
Single-cell metabolomics aims to gain a better understanding of how cells deal with environmental or chemical stress at a molecular level. It can also show how cells function individually, as well as give more understanding of ageing, cancer, or drug resistance at a molecular level.
There are currently four main methods to quantify the metabolome in single cells:
- Fluorescence-based detection
- Fluorescence biosensors
- FRET (Förster resonance energy transfer) biosensors
- Mass spectroscopy
Fluorescence-based approaches are able to detect different molecules in cells. Fluorescent proteins can act as metabolite detectors, emitting light when they bind to specified metabolites. For non-single cell analysis, researchers can add fluorescent tags to specified molecules – this is too invasive to study the metabolome in single cells as it alters the activity of the cell metabolites.
Mass spectroscopy is emerging as the key player in single-cell metabolomics. It can detect metabolites present in femtomole amounts (one billionth of a millionth of a mole, 10-15). Mass spectroscopy also does not need fluorescent proteins in order to work.
Challenges For Single-Cell Analysis
Single-cell analysis is not without its challenges, however.
- Data sparsity
Single-cell analysis with RNA-seq has more associated noise than larger cell population analysis with the same technology. Significant distortions of the ‘omics data arise from the small amount of starting material which is then hugely amplified. Transcripts that are expressed at low levels in some cells may seem to be abundant due to the amplification methods. These errors can artificially inflate cell-cell variability unnecessarily.
- Cell population heterogeneity
Cell populations are not uniform. Factors such as cell size or cycle state can pose challenges to data calibration or interpretation as they cause slight variation in the ‘omics data received from different single cells – even when the cells are part of the same population.
- Lack of context
Isolating single cells removes them from their usual environment. By looking at any ‘omics data for a single cell by itself, we may lose the contextual information about the cell’s spatial environment and dynamic position. These external information sources can help to better interpret a cell’s state at its isolation point.
Conclusion
Single-cell analysis allows us to draw information from individual cells rather than just a homogenised cell population. As each cell has a distinct lineage and ‘omic dataset, this can change how they respond to their environment.
It will help to better understand the nature and complexity of different diseases, and potentially unlock more effective therapies. However, researchers cannot overlook the challenges of single-cell analysis and must remember to properly detect the true heterogeneity in cell populations.
Fios offers a wide range of data analysis solutions, including for metabolomics and proteomics data specifically. For more information about how Fios can help with your ‘omics data needs, see the services we offer.
Author: Breige McBride, Content and Social Media Manager, Fios Genomics
Reviewed by Fios Genomics Bioinformatics Experts to ensure accuracy
References:
Challenges and emerging directions in single-cell analysis
Single-cell analysis: Advances and future perspectives
Microfluidic hydrodynamic trapping for single cell analysis: mechanisms, methods and applications
You may also be interested in:
Single-Cell vs Bulk RNA Sequencing
Leave a Reply
You must be logged in to post a comment.